Internet Locator
In today's digitally connected world, the ability to locate and navigate through various online platforms has become an essential skill. With the advent of Internet Locator, individuals and businesses can now effortlessly explore the vast online landscape. In this blog post, we will delve into the concept of Internet Locator, its significance, and how it has revolutionized how we navigate the digital realm.
Routing table growth: There has been exponential growth in Internet usage, and the scalability of today's Internet routing system is now a concern. With more people surfing the web than ever, the underlying technology must be able to cope with demand.
Whereas in the past, getting an internet connection via some internet locator service could sometimes be expensive, nowadays, thanks to bundles that include telephone connections and streaming services, connecting to the web has never been more affordable. It is also important to note that routing table growth has a significant drive driving a need to reexamine internet connectivity.
Limitation in technologies: This has been met with the limitations and constraints of router technology and current Internet addressing architectures. If we look at the core Internet protocols that comprise the Internet, we have not experienced any significant change in over a decade.
The physical-layer mechanisms that underlie the Internet have radical changed, but only a small number of tweaks have been made to BGP and its transport protocol, TCP. Mechanisms such as MPLS were introduced to provide a workaround to IP limitations within the ISP. Still, Layer 3 or 4 has had no substantial change for over a decade.
Matt Conran
Highlights: Internet Locator
Path Selection
In the Forwarding Information Base (FIB), prefix length determines the path a packet should take. Routing information bases (RIBs), or routing tables, program the FIB. Routing protocol processes present routes to the RIB. Three components are involved in path selection:
- In the subnet mask, the prefix length represents the number of leading binary bits in the on position.
- An administrative distance rating (AD) indicates how trustworthy a routing information source is. If a router learns about a route to a destination from multiple routing protocols, it compares the AD.
- Routing protocols use metrics to calculate the best paths. Metrics vary from routing protocol to routing protocol.
Prefix Length
Here’s an example of how a router selects a route when the packet destination falls within the range of multiple routes. Consider a router with the following routes, each with a different prefix length:
- 10.0.3.0/28
- 10.0.3.0/26
- 10.0.3.0/24
There are various prefix lengths (subnet masks) for these routes, also known as prefix routes. RIBs, also known as routing tables, contain all of the routes that are considered different destinations. Unless the prefix is connected to a network, the routing table includes the outgoing interface and the next-hop IP address.
Related: Before you proceed, you may find the following posts helpful:
- Container Based Virtualization
- Observability vs Monitoring
- Data Center Design Guide
- LISP Protocol
- What Is BGP Protocol In Networking
Internet Locator
The Internet is often represented as a cloud. However, this needs to be clarified as there are few direct connections over the Internet. The Internet is also a partially distributed network. It is decentralized, with many centers or nodes and direct or indirect links. There are also different types of networks on the Internet. For example, we have a centralized, decentralized, and distributed network.
The Internet is a conglomeration of independent systems representing organizations’ administrative authority and routing policies. Autonomous systems are made up of Layer 3 routers that run Interior Gateway Protocols (IGPs) such as Open Shortest Path First (OSPF) and Intermediate System-to-Intermediate System (IS-IS) within their borders and interconnect via an Exterior Gateway Protocol (EGP). The current Internet de facto standard EGP is the Border Gateway Protocol Version 4 (BGP-4), defined in RFC 1771.
1st Lab guide on BGP
In the following, we see a simple BGP design. BGP operated over TCP, more specifically, TCP port 179. BGP peers are created and can be iBGP or EBGP. In the screenshots below, we have an iBGP design. Remember that BGP is a Path Vector Protocol and utilizes a path vector protocol, which considers various factors while making routing decisions. These factors include the number of network hops, network policies, and path attributes such as AS path, next-hop, and origin.
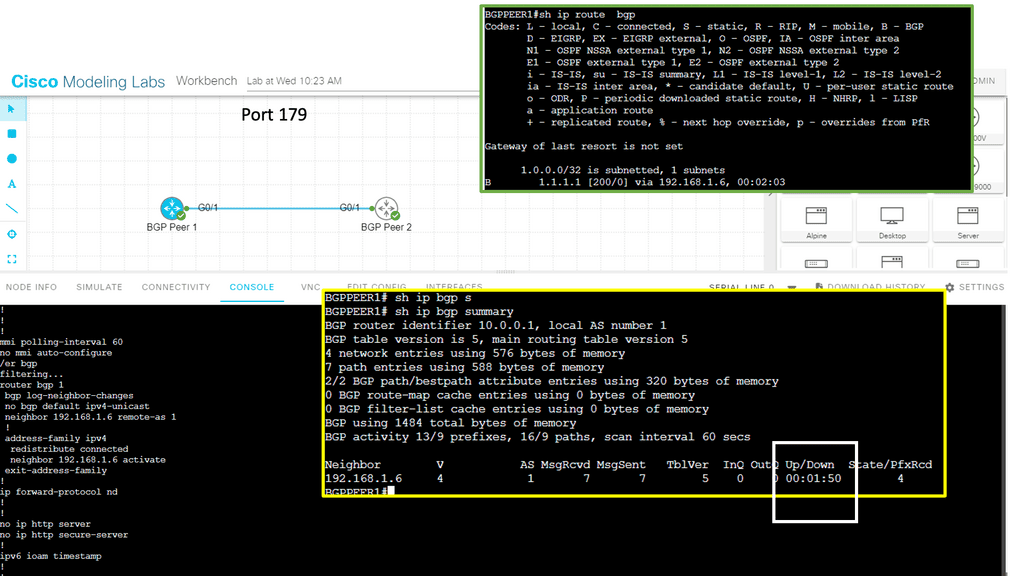
1. Path Vector Protocol: BGP utilizes a path vector protocol, which considers various factors while making routing decisions. These factors include the number of network hops, network policies, and path attributes such as AS path, next-hop, and origin.
Internet Locator: Default Free Zone ( DFZ )
The first large-scale packet-switching network was ARPAnet- the modern Internet’s predecessor. It used a simplex protocol called Network Control Program ( NCP ). NCP combined addressing and transport into a single protocol. Many applications were built on top of NCP, which was very successful. However, it lacked flexibility. As a result, reliability was separated from addressing and packet transfer in the design of the Internet Protocol Suite, with IP being separated from TCP.
On the 1st of January 1983, ARPAnet officially rendered NCP and moved to a more flexible and powerful protocol suite – TCP/IP. The transition from NCP to TCP/IP was known as “flag day,” It was quickly done with only 400 nodes to recompute.
Today, a similar flag day is impossible due to the sheer size and scale of the Internet backbone. The requirement to change anything on the Internet is driven by necessity, and it’s usually slow to change such a vast network. For example, inserting an additional header into the protocol would impact IP fragmentation processing and congestion mechanism. Changing the semantics of IP addressing is problematic as the IP address has been used as an identifier to higher-level protocols and encoded in the application.
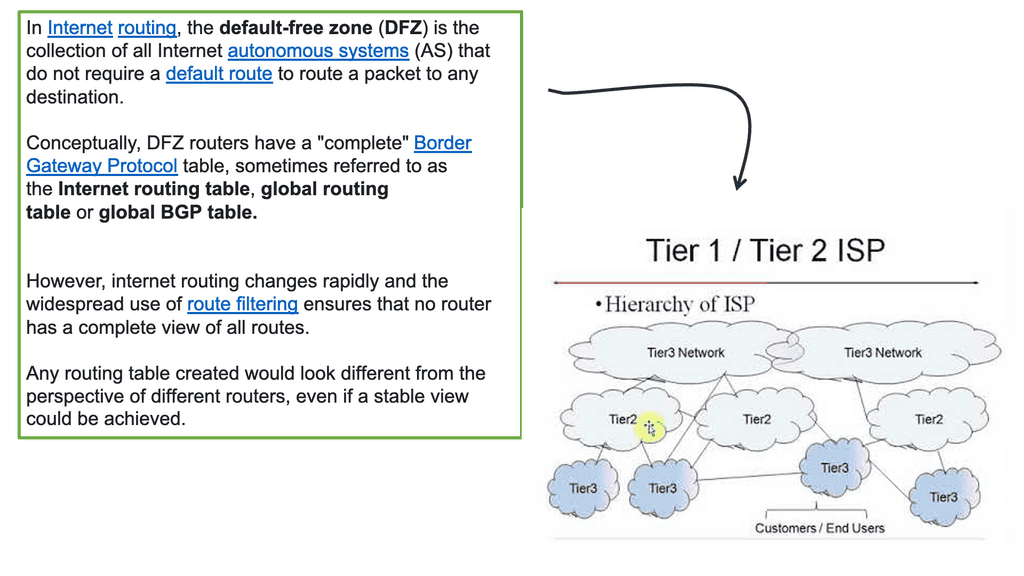
The driving forces of the DFZ
Many factors are driving the growth of the Default Free Zone ( DFZ ). These mainly include multi-homing, traffic engineering, and policy routing. The Internet Architecture Board ( IAB ) met on October 18-19th, 2006, and their key finding was that they needed to devise a scalable routing and addressing system. Such an addressing system must meet the current challenges of multi-homing and traffic engineering requirements.
Internet Locator: Locator/ID Separation Protocol ( LISP )
There has been some progress with the Locator/ID separation protocol ( LISP ) development. LISP is a routing architecture that redesigns the current addressing architecture. Traditional addressing architecture uses a single name, the IP address, to express two functions of a device.
The first function is its identity, i.e., who, and the second function is its location, i.e., where. LISP separates IP addresses into two namespaces: Endpoint Identifiers ( EIDs ), non-routable addresses assigned to hosts, and Routing Locators ( RLOCs), routable addresses assigned to routers that make up the global routing system.
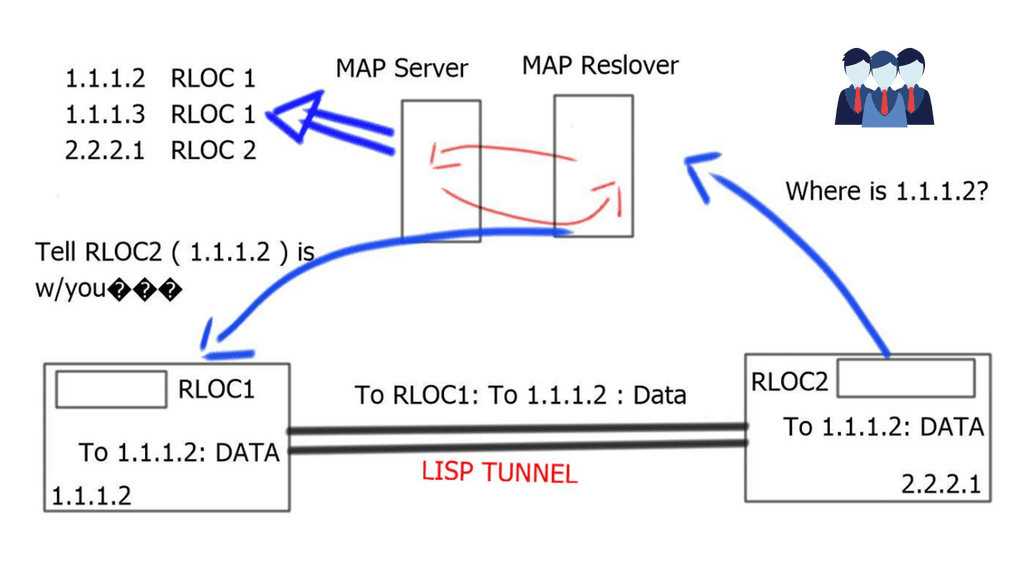
Separating these functions offers numerous benefits within a single protocol, one of which attempts to address the scalability of the Default Free Zone. In addition, LISP is a network-based implementation with most of the deployment at the network edges. As a result, LISP integrates well into the current network infrastructure and requires no changes to the end host stack.
2nd Lab guide on LISP.
In the following guide, we will look at a LISP network. These LISP protocol components include the following:
- Map Registration and Map Notify.
- Map Request and Map-Reply.
- LISP Protocol Data Path.
- Proxy ETR.
- Proxy ITR.
LISP implements the use of two namespaces instead of a single IP address:
- Endpoint identifiers (EIDs)—assigned to end hosts.
- Routing locators (RLOCs) are assigned to devices (primarily routers) that comprise the global routing system.
Splitting EID and RLOC functions yields several advantages, including improved routing system scalability, multihoming efficiency, and ingress traffic engineering. With the command: show lisp site summary, site 1 consists of R1, and site 2 consists of R2. Each of these sites advertises its own EID prefix. On R1, the tunnel router, we see the routing locator address 10.0.1.2. The RLOCs ( routing locators ) are interfaces on the tunnel routers.
Border Gateway Protocol (BGP) role in the DFZ
Border Gateway Protocol, or BGP, is an exterior gateway protocol that allows different autonomous systems (AS) to exchange routing information. It is designed to enable efficient communication between different networks and facilitate data exchange and traffic across the Internet.
Exchanging NLRI
BGP is the protocol used to exchange NLRI between devices on the Internet and is the most critical piece of Internet architecture. It is used to interconnect Autonomous systems on the Internet, and it holds the entire network together. Routes are exchanged between BGP speakers with UPDATE messages. The BGP routing table ( RIB ) now stands at over 520,000 routes.
Although some of this growth is organic, a large proportion is driven by prefix de-aggregation. Prefix de-aggregation leads to increased BGP UPDATE messages injected into the DFZ. UPDATE messages require protocol activity between routing nodes, which requires additional processing to maintain the state for the longer prefixes.
Excess churn exposes the network’s core to the edges’ dynamic nature. This detrimental impacts routing convergence since UPDATES need to be recomputed and downloaded from the RIB to the FIB. As a result, it is commonly viewed that the Internet is never fully converged.
Security in the DFZ
Security is probably the most significant Internet problem; no magic bullet exists. Instead, an arms race is underway as techniques used by attackers and defenders co-evolve. This is because the Internet was designed to move packets from A to B as fast as possible, irrespective of whether B wants any of those packets.
In 1997, a misconfigured AS7007 router flooded the entire Internet with /24 BGP routes. As a result, routing was globally disrupted for more than 1 hour as the more specific prefixes took precedence over the aggregated routes. In addition, more specific routes advertised from AS7007 to AS1239 attracted traffic from all over the Internet into AS1239, saturating its links and causing router crashes.
There are automatic measures to combat prefix hijacking, but they are not widely used or compulsory. The essence of BGP design allows you to advertise whatever NLRI you want, and it’s up to the connecting service provider to have the appropriate filtering in place.
Drawbacks to BGP
BGP’s main drawback concerning security is that it does not hide policy information, and by default, it doesn’t validate the source. However, as BGPv4 runs over TCP, it is not as insecure as many think. A remote intrusion into BGP would require guessing the correct TCP numbers to insert data, and most TCP/IP stacks have hard-to-predict TCP sequence numbers. To compromise BGP routing, a standard method is to insert a rogue router that must be explicitly configured in the target’s BGP configuration as a neighbor statement.
Significance of BGP:
1. Inter-Domain Routing: BGP is primarily used for inter-domain routing, enabling different networks to communicate and exchange traffic across the internet. It ensures that data packets reach their intended destinations efficiently, regardless of the AS they belong to.
2. Internet Service Provider (ISP) Connectivity: BGP is crucial for ISPs as it allows them to connect their networks with other ISPs. This connectivity enables end-users to access various online services, websites, and content hosted on different networks, regardless of geographical location.
3. Redundancy and Load Balancing: BGP’s dynamic routing capabilities enable network administrators to create redundant paths and distribute traffic across multiple links. This redundancy enhances network resilience and ensures uninterrupted connectivity even during link failures.
4. Internet Traffic Engineering: BGP plays a vital role in Internet traffic engineering, allowing organizations to optimize network traffic flow. By manipulating BGP attributes and policies, network administrators can influence the path selection process and direct traffic through preferred routes.